Shape-preserving Chemical Conversion
Key Research Questions
This research thrust is focused on developing a better understanding of the fundamental mechanisms associated with the shape-preserving chemical conversion of biosilica structures into new, technically-important functional materials. The following basic research questions are being addressed in this thrust:
- How do the nanostructure and nanochemistry of biogenic silica preforms evolve during the course of chemical conversion?
- How do conversion conditions (temperature, reactant partial pressure, reactant flow rate, silica micro/nanostructure, etc.) affect such evolution?
- What are the rate-limiting steps associated with such chemical conversion?
- What are the key factors that affect the retention of shape and fine features upon chemical conversion?
Experimental Approaches
In this research thrust, the BaSIC (Bioclastic and Shape-preserving Inorganic Conversion) process is being used to convert biogenic silica preforms into new functional compositions. Chemical conversion of diatom microshells (naturally-occurring or genetically-modified) and biosculpted silica preforms (synthetically-patterned, silaffin-derived structures) is being conducted inside furnaces operating with computer-controlled thermal schedules and regulated gas atmospheres. The reaction conditions within such furnaces are monitored with calibrated thermocouples and gas sensors.
Electron microscopy and diffraction analyses are being used to examine the evolution of:
- microstructure (i.e., external structure: preform shape and
dimensions),
- nanostructure (i.e., internal structure: types, amounts, sizes, and distributions of internal phases), and
- nanochemistry (i.e., internal chemistry: compositions of phases formed throughout preform cross-sections)
associated with such chemical conversion. Small changes in the shapes and fine features of biogenic silica preforms upon reactive conversion are being evaluated with scanning electron microscopic (SEM) analyses. Changes in internal structure and chemistry at the nanoscale are being determined by transmission electron microscopic (TEM) analyses of ion milled cross-sections. Local electron diffraction (ED) and energy-dispersive x-ray (EDX) analyses of partially-converted and fully-converted specimens are being used to precisely evaluate phase evolution and reaction paths. High-temperature x-ray diffraction (HTXRD) analyses are also being used for dynamic evaluation of global changes in structure and chemistry during reactive conversion. Dynamic HTXRD analyses, and static electron microscopic analyses of partially-reacted specimen cross-sections, are being used to quantify the extent of conversion as a function of time, temperature, reactant gas partial pressures, and reactant flow rates.
MURI Collaborators
The major MURI participants are:
The technical expertise of each of these collaborators is well-suited for this research thrust.
Professor Sandhage is the inventor of the BaSIC process and several other near net-shape ceramic conversion processes involving high-temperature gas/solid or liquid/solid reactions. His other related research interests include: i) ceramics and composites for aerospace, sensor, electromagnetic, biomedical, and refractory applications, ii) high-temperature inorganic chemistry (e.g., interactions between ceramics and reactive fluids), and iii) high-temperature corrosion of ceramics and metals.
Professor Snyder is a recognized expert in x-ray analyses and the crystallography of ceramic materials. Prof. Snyder will be using state-of-the-art x-ray analytical equipment for evaluating the phase evolution and transformation kinetics of 3-D bioclastic and biosculpted preforms into new compositions. Dr. Snyder and Dr. Sandhage have developed a new type of heating stage for high-temperature x-ray diffraction that allows for real-time kinetic analyses with controlled, reactive atmospheres.
Professor Rapp has developed strong expertise, over the past four decades, on the thermodynamic and kinetic aspects of high-temperature gas/solid and solid/solid reactions. His other related research interests include: i) diffusion coatings and evaporation phenomena, ii) solid-state electrochemistry and solid-state reactions, iii) high-temperature corrosion, and iv) the electrochemistry of fused salts.
In addition to the main MURI investigators for this thrust, collaborations with other MURI participants will be utilized for: i) obtaining bioclastic structures for this thrust (e.g., native and genetically-modified diatom microshells from M. M. Hildebrand and B. P. Palenik), ii) providing biosculpted structures for this thrust (e.g., synthetically-patterned, silaffin-derived silica preforms from R. R. Naik, M. O. Stone, and D. J. Hansford), and iii) incorporating the chemically-modified structures generated in this thrust into microdevices and the testing of such devices (D. J. Hansford, A. T. Conlisk, Jr.).
Prior Related Work by MURI Collaborators
Recent work by MURI collaborators (Sandhage, Naik, Stone) has shown that diatom frustules (bioclastic silica) and biosculpted silica microfilaments can be converted into other ceramics with a preservation of the biosilica shape and fine (102 nm) features. This prior work involved the following gas/silica displacement (oxidation-reduction) reaction:
2Mg(g) + SiO2(s) => 2MgO(s) + {Si} [1]
In this reaction, {Si} refers to silicon present in a Mg-Si liquid that formed by continued reaction of elemental Si (produced upon reduction of SiO2) with excess Mg gas. Secondary electron images of the same diatom microshells before and after such reactive conversion are shown in Figures 1a and b, respectively. These diatom microshells (Aulacoseira) possessed a hollow cylinder shape with fine (102 nm) pores running in rows along the cylinder length. Comparison of Figures 1a and b reveals that the overall shape and fine features of the diatom microshells were retained upon conversion into MgO (note: the formation of MgO was confirmed by x-ray diffraction and energy-dispersive x-ray, EDX, analyses). Ten specific surface features were clearly observed, along with the fine pores, before and after reaction. The solidified Mg-Si product of reaction [1] can be seen below the reacted microshell in Figure 1b (the Mg-Si liquid poured out of the converted MgO microshell). Image analyses indicated that there was no significant difference in the size distributions of the fine pores on the microshell walls before and after reaction with Mg(g). A higher magnification image, revealing the nanocrystalline nature of the converted microshell, is shown in Figure 1c. A transmission electron image of a cross-section of a converted MgO microshell is shown in Figure 1d. TEM/EDX analyses at various locations throughout this cross-section yielded peaks for Mg and O, but not Si, which was consistent with complete migration of the Mg-Si liquid reaction product away from the MgO-converted microshell.
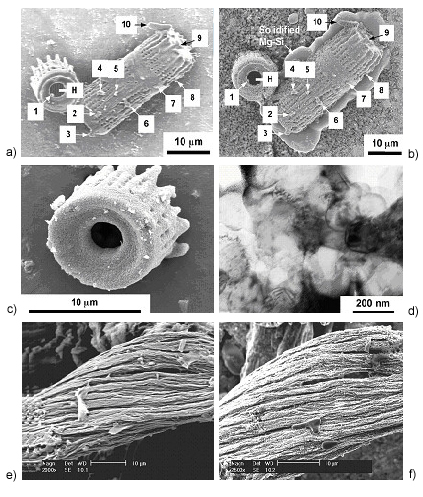 |
Figure 1. Secondary electron images of: a) diatom microshells (bioclastic silica), b) the same microshells in a) after conversion into MgO by reaction with Mg(g) at 900°C (reaction [1]), c) a MgO-converted microshell at higher magnification, e) microfilamentary (biosculpted) silica generated with the use of a silaffin-derived peptide, and f) the same microfilamentary specimen after reaction with Mg(g) for 4 h at 900°C. A transmission electron image of a cross-section of a converted MgO microshell is shown in d).
Patterned bioderived structures with tailored (non-natural) shapes have also been converted into other oxides via the BaSIC process. Stone, Naik, et al. (AFRL/WPAFB) have synthesized microfilamentary biosilica structures by passing a mixture of a silaffin-derived peptide (a 19-amino-acid segment of the silaffin-1 polypeptide from the diatom Cylindrotheca fusiformis) with a silicic acid solution in a laminar flow pattern. Such microfilamentary silica is shown in Figure 1e. This silica preform was exposed to Mg(g) for 4 h at 900°C. The resulting MgO-converted specimen is shown in Figure 1f. The general shape and microfilamentary nature were retained upon conversion into MgO. These images demonstrate that “biosculpted” nanoparticle structures (that is, nanoparticle structures with shapes that are obtained through the patterning of biomolecules) may be converted into other oxides with a retention of the starting shapes and fine features. This novel biosculpting and reactive conversion approach opens the door to a wider variety of ceramic shapes and compositions than are available in nature, so that a broader range of potential microdevices may be produced for DoD applications.
While the feasibility of the BaSIC conversion method for producing near net-shaped, chemically-converted micro/nanostructures from biosilica preforms has been demonstrated, fundamental understanding of the basic kinetic mechanisms and nanostructural evolution associated with such conversion is lacking. Key criteria required for preservation of biosilica structures upon complete reaction are also not known. By understanding how to manipulate processing conditions for optimized rates of conversion, the precision with which meso-to-nanoscale features can be retained upon conversion of complex-shaped bioclastic/biosculpted preforms should be enhanced (e.g., with a high rate of conversion at low temperatures, the extent of sintering or grain growth during conversion may be minimized). A wider range of conversion reactions also needs to be examined, to open the door to a wider variety of biologically-derived micro/nanocomponents.
Return to top
|